Deficiency of iodine, as a
primitive antioxidant, seems to cause more damage in developing embryos
than some other “modern” antioxidants. In fact, in pregnant women
I-deficiency causes abortions and stillborns (Dunn and Delange 2001).
Molecular iodine (I2) has the chemical capacity to non-specifically
iodinate amino acids, proteins and lipids (Gottardi 1991). The oxidation
of iodide by reactive oxygen species (ROS) like H2O2 has been studied
since the early 1920s, and it is a necessary step to incorporate iodine
into bioactive molecules (Bray and Caulkins 1921). These reactions yield a
complex mixture of different iodine species. One factor that contributes
to this complexity is the range of oxidation states associated with iodine
species: -1 to +5, e.g. -1 (iodide – I-); +1 (hypoiodic acid - HOI); +5 (iodate
- IO3) as shown in Table 1.

Table. 1. From Gottardi, 1991
Several oxidized iodine species formed from
iodide oxidation have the potential to react with both water and I- (Bray
and Caulkins, 1921). The iodine species that exist at a pH 7.4 are: iodide
(I-), triiodide (I3 ), molecular iodine (I2), hypoiodious acid (HOI),
hypoiodite ion (OI-) and the iodine anion (HI2O-). In 1985, Venturi
suggested that the antioxidant biochemical mechanism of iodides was
probably one of the most ancient mechanisms of defense from poisonous ROS
as shown in Table 2 and in Table 3.
2 I- à I2 + 2 e- (electrons) = -
0.54 Volt ;
2 I- + Peroxidase + H2O2 + 2
Tyrosine à 2 Iodo-Tyrosine + H2O + 2 e- (antioxidants);
2 e- + H2O2 + 2 H+ (of
intracellular water-solution) à 2 H2O
Table. 2. Proposed
antioxidant biochemical mechanism of iodides (From Venturi 1985)
2 I- + Peroxidase + H2O2 + Tyrosine,
Histidine, Lipids, Carbons à
à Iodo-Compounds +
H2O + 2 e- (antioxidants)
Iodo-Compounds: Iodo-Tyrosine,
Iodo-Histidine, Iodo-Lipids, Iodo-Carbons
Table. 3. Proposed
antioxidant biochemical mechanism of iodides, probably one of the most
ancient mechanisms of defense from poisonous reactive oxygen species
(Modified from Venturi 2003).
Petersén
et al. (1996), Küpper et al. (1998, 2002) and Gall et al. (2004) suggested
that the production of volatile iodo-compounds by marine algae is a result
of the development of photosynthesis, oxygen production and respiration
some 3 billion years ago, and it is due to adaptation to light in order to
reduce the amount of poisonous ROS, such as hydrogen peroxide, superoxide
radicals and hydroxyl radicals.
Increase of Oxygen in Earth’s
Atmosphere and its Biological Consequences
The evolution of
oxygen-producing cells was probably the most significant event in the
history of life after the beginning of life itself. Oxygen is a potent
oxidant whose accumulation into the atmosphere forever changed the surface
chemistry of Earth (Canfield 2005). Lane (2002), Wiedenheft et al. (2005)
and Benzie et al. (2003) suggested that the evolution of oxygenic
photosynthesis marks the dawn of oxidative stress and represents one of
the greatest selective pressures imposed on primordial life. The
association of molecular oxygen with abundant ferrous iron pools produced
two major biological consequences. First, life dependent on the redox
properties of Fe(II) had to contend with its oxidation and precipitation
as Fe(III). Secondly, life had to contend with the toxicity of ROS
generated by the partial reduction of dioxygen by ferrous iron. By the
start of the Cambrian period 570 million years ago, or somewhat earlier,
oxygen levels had apparently increased enough to permit rapid evolution of
large oxygen-utilizing multicellular organisms. ROS potentially react with
lipids, proteins, carbohydrates and DNA and thus interfere with the
functions of cellular membranes, cell metabolism, cellular signaling, cell
growth and differentiation. Oxidative stress seems to have been implicated
as a causative process in the development of a vast number of degenerative
diseases (Suzuki et al. 1997; Flohe et al. 1997; Yu 1994; Sies 1997).
Stone (1988) studied the role of the primitive sea in the natural
selection of iodides as a regulating factor in inflammation. This author
reported that iodides have many non-endocrine biologic effects, including
a role they play in the physiology of the inflammatory response. Iodides
increase the movement of granulocytes into areas of inflammation and
improve the phagocytosis of bacteria by granulocytes and the ability of
granulocytes to kill bacteria.
Early Developments in
Antioxidant Defense
During
evolution, endogenous protection systems have developed to counteract the
deleterious effects of cellular oxidation. Protective antioxidant enzyme
systems consist primarily of superoxide dismutase, glutathione peroxidase,
catalase and peroxiredoxins. In addition to these endogenous systems,
exogenous dietary antioxidants may help to prevent oxidative stress. In
particular, mineral antioxidants present in the primitive sea, as some
reduced compounds of Rubidium, Vanadium, Zinc, Iron, Cuprum, Molybdenum,
Selenium and Iodine (I), which play an important role in electron transfer
and in redox chemical reactions. Most of these substances act in the cells
as essential trace-elements in redox and antioxidant metallo-enzymes.
Some researchers hypothesized that the relative composition of many
mineral trace-elements of the animal body is similar to the composition of
the primitive sea, where the first forms of life began (Favier 1991).
During evolution, in the last 500-400 million years (My) antioxidants of
terrestrial origin developed in plants as many polyphenols, carotenoids,
flavonoids, tocopherols and ascorbic acid. A few of these appeared more
recently, in last 200-100 My, in fruits and flowers of angiosperm plants (Venturi
2004, 2006). In fact Angiosperms (the dominant type of plant today) and
most of their antioxidant pigments evolved during the late Jurassic
period. Plants employ antioxidants to defend their structures against ROS
produced during photosynthesis. The human body is exposed to the very same
oxidants, and it has also evolved an effective antioxidant system.
Plant-based, antioxidant-rich foods traditionally formed the major part of
the human diet, and plant-based dietary antioxidants are hypothesized to
have an important role in maintaining human health. Benzie (2000, 2003)
reported that the estimated daily intake of many selected antioxidants
(such as antioxidant vitamins, polyphenols, carotenoids and flavonoids)
decreased quantitatively from palaeolithic to modern
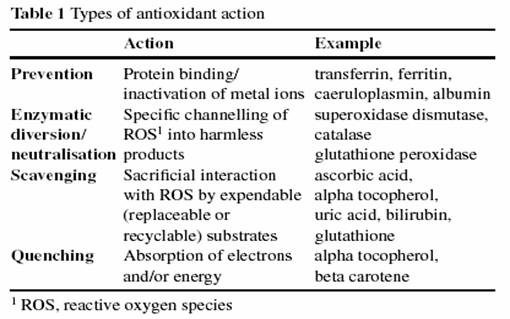
Iodide/iodine and Iodide/thyroxine:
Evolutionary History of a Primitive AntioxidantOver
three billion years ago, blue-green algae were the most primitive oxygenic
photosynthetic organisms, ancestors of multicellular eukaryotic algae.
Algae that contain the highest amount of iodine (1-2 % of dry weight) and
peroxidase enzymes, were the first living cells to produce poisonous
oxygen in the atmosphere (Obinger et al. 1997a, b; Venturi et al. 2000a,
b). Therefore Venturi suggested that algal cells required a protective
antioxidant action of their molecular components, in which iodides,
through peroxidase enzymes, seem to have had this specific role (Venturi
1985; Venturi et al. 1987, 1993, 1999). In fact iodides are greatly
present and available in the sea, where algal phytoplankton, the basis of
marine food-chain, acts as a biological accumulator of iodides, selenium
(and n-3 fatty acids) (Cocchi and Venturi 2000). The sea is rich in
iodine, about 60 micrograms (mg) per liter, since this is where most of
the iodine removed and washed away from the soil accumulated due to rains
and the glacial ages (Elderfield and Truesdale 1980) (Fig. 1).
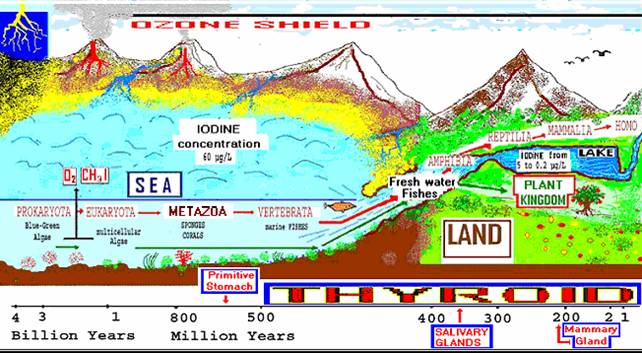
Figure 1. IODINE and
EVOLUTION.
Over three billion years ago,
blue-green algae were the first living Prokaryota to produce oxygen and
emit volatile halocarbons and CH3I in the atmosphere. For 700 million
years, thyroxine has been present in fibrous exoskeletal scleroproteins of
the lowest invertebrates. About 500-400 million years ago (Mya) some
primitive marine fishes began to emerge from the iodine-rich sea and
transferred to iodine-deficient terrestrial fresh waters. 400-300 Mya some
vertebrates evolved in amphibians and reptiles and transferred to
I-deficient land. Then, from primitive gastro-intestinal cells, a new
“thyroidal” follicular organ developed, as a reservoir for iodine. In
vertebrates, thyroid hormones became active in the metamorphosis and
thermogenesis for a better adaptation to terrestrial environment. (From
Venturi 2004).
The major iodine species in
sea waters are iodate and iodide, along with smaller concentrations of
molecular iodine, hypoiodous acid and iodinated organic compounds (Truesdale
et al. 1995). Brown algae (seaweeds) accumulate iodine to more than 30,000
times the concentration of this element in seawater (Colin et al. 2003;
Teas et al. 2004). Not much is known, however, on the iodine-concentrating
mechanisms and on the biological functions of iodine in algae. Primitive
marine prokaryotes seem to have an efficient active “iodide pump”,
ancestor of the pump of multicellular eukaryotic algae and of mammalian
iodide transporters. The mechanism of “iodide-pump” in the cells is very
ancient and lacking of specificity, in fact, it is not able to distinguish
iodide from other anions of similar atomic or molecular size, which may
act as “pseudo-iodides”: thiocyanate, cyanate, nitrate, pertechnate,
perchlorate (Wolff 1964). It is hypothesized that 80% of the Earth's
oxygen is produced by planktonic algae, prochlorphytes, cyanobacteria and
the free-floating unicellular microbes inhabiting the sea close to the
surface. Up till now only one aspect of halogen metabolism, the production
of volatile halocarbons, seems to have attracted more attention from
researchers, because these compounds, and in particular the iodinated
forms, have a significant impact on the chemistry of the atmosphere, and
its ozone shield depletion (Carpenter et al. 1999, 2000). Halogen
metabolism in marine algae involves enzymes known as haloperoxidases,
which catalyse the oxidation of halides into hypohalous acids (Vilter et
al. 1983; Vilter 1994, 1995; Gribble 1996; Pedersèn et al. 1996; Dembitsky
et al. 2003; Gall et al. 2004). Since iodoperoxidase of Laminaria seaweeds
is more efficient than the bromoperoxidase in the oxidation of iodide
(Colin et al. 2003), this former activity may be more largely responsible
for the uptake of iodide from seawater. There is an increased emission of
iodinated halocarbons both from kelp beds at low tide during day-time
(Carpenter et al. 1999, 2000), and from kelp plants incubated under high
solar irradiance, caused by photo-oxidative stress, compared to plants
kept in the shade (Gall et al. 2004). The green algae are hypothesized to
have been ancestors of terrestrial plants. Recently Berking et al. (2005)
confirmed Venturi’s hypothesis concerning antioxidant iodide and thyroxine
in some marine invertebrates (polyps of the jellyfish Aurelia aurita)
which contain iodide ions. Berking reported that in these invertebrates
“the danger to be harmed by iodine is strongly decreased by endogenous
tyrosine which reacts with iodine to form iodiferous tyrosine compounds
including thyroxin. Both substances together, iodide and tyrosine, form an
efficient oxidant defense system which shields the tissue against damage
by ROS.” Spangenberg (1971) observed that when polyps of Aurelia are
maintained for some weeks in iodide-free surroundings, it is possible to
cause strobilation (metamorphosis) by applying iodine compounds including
T4. (Polyps kept in normal seawater do not respond to strobilation). The
interesting observation was that the polyps underwent strobilation when
iodide was applied for 24 hours. But a 24-hour treatment with T4 was not
sufficient. The treatment with T4 must carried out over a longer period of
time to induce strobilation. It appears that T4 can deliver iodide, but
this requires time. Recently Heyland and Moroz (2005) and Heyland et al
(2006) reported that the oxidation of iodide to iodine in some marine
invertebrates is a critical step for scavenging ROS, and that the reaction
of iodine with tyrosine residues removes potentially poisonous iodine from
the cell. In vertebrates, isolated cells of extrathyroidal
iodide-concentrating tissues can produce protein-bound mono-iodo-tyrosine
(MIT), di-iodo-tyrosine (DIT) and also some iodolipids (Banerjee et al.
1985; Aceves et al. 2005). This pathway for iodine organification involves
iodine incorporation into specific lipid molecules. Iodolipids have been
shown to be regulators of mammalian cellular metabolism. Iodine, reacting
with double bonds of some polyunsaturated fatty acids of cellular
membranes, makes them less reactive with ROS (Cocchi and Venturi 2000).
Two iodinated lipids may be iodine autoregulation mediators:
6-iodo-5-hydroxy-8,11,14-eicosatrienoic acid (delta-iodo-lactone) and
2-iodohexadecanal. Delta-iodolactone has been found to be a potent
inhibitor of proliferation of thyroidal and of some non-thyroidal cells (Banerjee
et al.1985; Pisarev et al. 1988; Dugrilllon 1996; Venturi et al. 2000a, b;
Cocchi and Venturi 2000; Cann et al. 2000; Aceves et al. 2005). According
to Aceves et al. (2005) the percentage of iodine in cellular homogenate of
breast tissue is about 40 % in lipid fraction and 50 % in protein
fraction. Aceves also reported that in mammary gland homogenates from
virgin rats, the addition of iodine in their diet significantly decreases
lipid peroxidation. The family of peroxidase enzymes includes mammal,
microorganism, plant, algal, and fungal peroxidases. Some of these
peroxidases, known as haloperoxidases, use halide ions (iodide, bromide,
and chloride) as natural electron donors, and have an antioxidant function
in Cyanobacteria (Obinger et al. 1997, 1999; Venturi and Venturi 1999).
Taurog (1999) reported that the relation between animal and non-animal
peroxidases probably represents an example of convergent evolution to a
common enzymatic mechanism. Heyland and Moroz (2005) suggest that
exogenous sources of thyroid hormones (THs) (from food) may have been
ancestral, while the ability to synthesize TH endogenously may have
evolved independently in a variety of metazoans, resulting in a diversity
of signaling pathways and, possibly, morphological structures involved in
TH-signaling. In fact, increasing evidence suggests that THs also function
in a variety of invertebrate species. The evidence of TH effects in
invertebrates has been reviewed in Eales (1997) and Heyland et al. (2005).
Evolution of Iodine from
Non-hormonal to Hormonal Functions
Since approximately 700-800
Mya thyroxine (T4) has been also present in fibrous exoskeletal
scleroproteins of the lowest marine invertebrates (sponges, corals) (Roche
1952; Roche and Yagi 1952). Recent studies reported that THs are also
present in unicellular planktonic alga (Dunaliella tertiolecta) and in
echinoid larvae (sea-urchin) (Chino et al. 1994; Heyland 2004). These
original sources of animal hormones might have been plants/algae in many
cases, and could well have been independently derived from plants/algae in
distinct lineages. The ancestral function of THs could also have been as
feeding deterrents in algae and/or plants and the signaling functions in
animals (Heyland and Moroz 2005; Eales 1997) might, therefore, have been
acquired secondarily, perhaps even through horizontal transfer from their
hosts or other co-associated microbes with more ancient relationships with
the host. In waters the iodine concentration decreases step by step from
sea-water to estuary (about 5 mg / L) and source of rivers (less than 0.2
mg / L in some Triassic mountain regions of northern Italy), and in
parallel, salt-water fishes (herring) contain about 500-800 mg of iodine
per kg compared to fresh-water trout about 20 mg per kg (Venturi and
Venturi 1999; Venturi et al. 2000a, b, 2003). So, in terrestrial
I-deficient fresh waters some trout and other salmonids (anadromous
migratory fishes) may suffer thyroid hypertrophy or related metabolic
disorders (Venturi et al. 2000a, b), as do some sharks in captivity.
Youson and Sower (2001) reported that iodide-concentrating ability of the
endostyle of sea lamprey was a critical factor in the evolution of
metamorphosis and that the endostyle was replaced by a follicular thyroid,
since post-metamorphic animals needed to store iodine following their
invasion of freshwater. According to Manzon and Youson (1997) in some
anadromous migratory fishes (sea lamprey and salmonids), iodine and TH
play a role in initiation of metamorphosis, which is induced by the
decline in serum of TH. After metamorphosis, when these adult marine
fishes die in fresh-water after reproducing, they release their iodides
and selenium, and n-3 fatty acids (Venturi et al. 2000a, b), in the
environment, where they have a favorable role in food for life and health
of native animals, bringing back upstream from the sea to I-deficient
areas these essential trace-elements (Venturi et al. 2000a, b). In some
I-deficient fresh-waters some salmonids may also suffers of “scurvy” and
spinal curvature, due to dietary vitamin C deficiency.
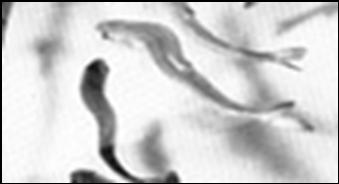
Fig. 2 . Cultured salmons in
freshwater showing nutritionally induced
spinal curvature (scoliosis
and lordosis) by vitamin C deficiency.
If these fishes are housed
in I-rich sea-water then this disease improve, presumably because of the
availability of other antioxidants in marine environment (Hardie et al.
1991). For this reason, we suggested that the antioxidant action of
ascorbic acid developed firstly in the plants when, about 500-400 Mya
plants began to adapting themselves to mineral (and iodine) deficient
estuaries of rivers and land. Some biologists suggested that many
vertebrates had developed their metabolic adaptive strategies in estuary
environment (Purves et al. 1998). The role of iodine in marine and
fresh-water fishes has not been completely understood, but it has been
reported that I-deficient fresh-water fishes suffer of higher incidence of
infective, parasitic, neoplastic and atherosclerotic diseases than marine
fishes. Farrell et al. (1992) reported the absence of coronary arterial
lesions in some elasmobranch fishes, living in I-rich sea-water. In
October 7, 1999, the U.S.A. Committee of the House and Senate regarding
"Marine Research" reported that " The Committee notes the unusually low
incidence of cancer in marine sharks, skates, and rays and encourages
basic research through the study of the immune system of these marine
animals and the examination of bioactive molecules from shark, skate, and
ray cells and tissues that have the potential to inhibit disease processes
in humans." Yun et al.(2005) reported that marine vegetation concentrates
iodine for its antimicrobial and antioxidant properties. In the
amphibians, environmental iodine is the essential metamorphosis-factor and
has an important role in the spectacular apoptosis on the cells of larval
gills, tail, fins and gastro-intestine, and in adapting and transforming
of aquatic animal (tadpole) to a “more developed” adult terrestrial animal
(frog). In fact, programmed cell death, with nuclear changes and removal
by phagocytic macrophages, occurs in a variety of organs, during amphibian
metamorphosis, that is under the control of iodinated TH. Indeed, because
of the massive cell death that occurs during a short period, amphibian
organs serve as an ideal model system for the study of mechanisms
underlying programmed cell death (Ishizuya-Oka et al. 2003; Ikuzawa et al.
2005).

Fig. 3. In amphibian
metamorphosis iodides and thyroxine have an important
role in the spectacular
apoptotic action on the cells of gills, tail and fins, and in
adapting and transforming an
aquatic animal (tadpole) into a “more developed”
terrestrial animal (frog).
TH induces apoptosis of
larval cells and differentiation of pepsinogen-producing cells in the
stomach of adult form of frog Xenopus laevis (Ishizuya-Oka et al. 2003).
Upadhyay et al. (2002) reported that excess iodine has been observed to
induce also apoptosis in thyrocytes and mammary cells. According to
Upadhyay, mitochondria are important in iodination of different proteins;
mitochondria are the central executioner of apoptosis and therefore may
play an important role in carcinogenesis. Mitochondrial proteins from
breast tissue are iodinated. Organification of iodine to proteins requires
oxidative enzymes, H2O2 generating system and proteins in the vicinity,
conditions favorable for the iodination of proteins, which exist in
mitochondria under normal circumstances. Upadhyay observed that
mitochondria isolated from the tumor (TT) and extra-tumoral tissue (ET) of
human breast display significant uptake of iodine. Mitochondrial proteins
were observed to be predominantly iodinated in ET but not in TT
mitochondria. Treatment with iodine showed an increase in mitochondrial
permeability transition of TT and decrease in ET. Iodine induced released
factors other than cytochrome c from tumor mitochondria, which initiated
apoptosis in vitro.
Iodine in Terrestrial
Organisms
When about 500-300 Mya some
living plants and animals began to transfer from the sea to rivers and
land, environmental I-deficiency was a challenge to the evolution of
terrestrial life (Venturi 2000a). In fishes, plants and animals the
terrestrial diet became deficient in many primitive marine trace-elements,
including iodine and selenium. Terrestrial plants slowly optimized the
production of “new” endogenous antioxidants such as ascorbic acid,
polyphenols, carotenoids, flavonoids, tocopherols, some of which became
essential “vitamins” in the diet of terrestrial animals (vitamins C, A,
E). According to Coic and Coppenet (1990) and Lamand (1991), iodine and
selenium became no longer necessary to many plants. In a different way,
some chordates, about 500 Mya, began to use also the “new” thyroidal
follicles, as reservoir for iodine, and to use the thyroxine in order to
transport antioxidant iodide and triiodothyronine into the peripherical
cells. Triiodothyronine (T3), the biologically active form of thyroid
hormone in vertebrates, became active in the metamorphosis and
thermogenesis for a better adaptation to terrestrial fresh-waters,
atmosphere, gravity, temperature and diet (Fig. 1). Extrathyroidal or
peripheral TH metabolism is mediated by deiodinases [type 1 deiodinase
(D1), type 2 deiodinase (D2) and type 3 deiodinase (D3)]. Deiodinases
deliver from iodothyronines into peripheral cells atoms of iodide without
hormonal action. D3 protein is also expressed by granulocytes and
monocarboxylate transporter 8 (MCT8), a novel very active and specific
thyroid hormone transporter is also present at the site of inflammation.
Thyroxine, reverse-T3 and iodothyronines seem to be important as
antioxidants and inhibitors of lipid peroxidation (Oziol et al. 2001;
Berking et al. 2005), and more effective than vitamin E, glutathione and
ascorbic acid (Tseng and Latham 1984). Moreover the new terrestrial diet
harbored plant iodide-transport inhibitors such as thiocyanates, cyanates,
nitrates and some glycosides (Wolff 1964; Brown-Grant 1961). Many plant
substances that inhibit iodide-transport seem to have antiparasitic
activity (Wolff 1964). In previous work, Venturi et al.(2000a) reported
that, contrary to amphibian metamorphosis, in the mammals the
thyroidectomy and hypothyroidism might be considered like a sort of
phylogenetical and metabolical regression to a former stage of reptilian
life. In fact, many disorders, similar to reptilian features, such as a
dry, hairless, scaly, cold skin and a general slowdown of metabolism,
digestion, heart rate, nervous reflexes with lethargic cerebration,
hyperuricemia, and hypothermia seem to afflict hypothyroid humans. The new
hormonal action was made possible by the formation of T3-receptors (TH-Rs)
in the cells of vertebrates. First, about 500 Mya, in marine chordates,
the primitive TH-Rs with a metamorphosing action appeared and then, about
250-350 Mya, in the birds and mammalians, others more recent TH-Rs with
metabolic and thermogenetic actions were formed. TH-R genes are indeed c-erbA
oncogenes, which have been implicated as tumor suppressor genes of
non-thyroidal cancers and are altered in some human gastric and mammary
cancer (Wang et al. 2002; Li et al. 2002).
Role of Iodide in Animal
Cells
In 2001, Hays reported in
“Thyroid ” journal that “It is surprising that the precise total iodine
content of the human body remains uncertain after many years of interest
in iodine metabolism … and that extra-thyroidal iodine pool remains a
matter of speculation and also the chemical nature non-thyroidal iodine
is unknown.”
In humans, the total amount
of iodine is about 25-50 mg and about 50-70 % of total iodine is
non-hormonal and it is concentrated, via NIS, in extrathyroidal tissues (Venturi
2000a, b).
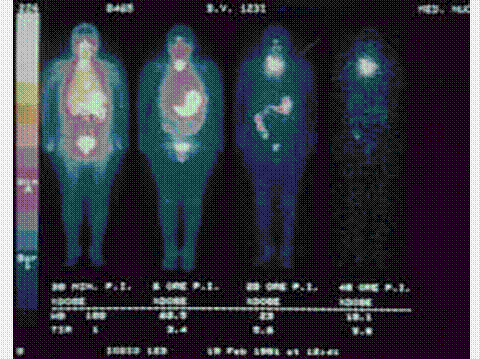
Figure 4. Sequence of I-123
total-body scintiscans of a woman after intravenous injection of I-123
(half-life: 13 hours); (from left) respectively at 30 minutes, and at 6,
20 and 48 hours. It is evident the highest and rapid concentration of
radio-iodide (in white) in gastric mucosa of the stomach, salivary glands
and oral mucosa. In gastric mucosa of the stomach, 131-I (half-life: 8
days) persists in scintiscans for more than 72 hours. In the thyroid
I-concentration is more progressive, as in a reservoir [from 1% (after
30 minutes) to 5.8 % (after 48 hours) of the total injected dose]. Mammary
gland iodide-concentration is here not evident because this woman was not
pregnant or lactating. It is evident a high excretion of radioiodide in
urina.
In 1996, cloning and
molecular characterization of the human NIS have been performed (Dai et
al. 1996; Smanik et al. 1996). In the thyroid cells active iodide
transport is facilitated by three transporters: NIS, Pendrin and Apical
Iodide Transporter. Expression of all three transporters appears in
extrathyroidal tissues (Burbridge 2005; Rodriguez et al. 2002). Iodine is
present, in different concentration, in every organ and tissue of the
human body, not just the thyroid gland. So far the list of these
iodide-concentrating cells includes: white blood cells, salivary and
lachrymal glands, ciliary body of the eye, renal cortex, the pancreas, the
liver, gastric, small and large intestinal mucosa, nasopharynx, choroid
plexus, skin, adrenal cortex, mammary gland, placenta, uterus and ovary
(Brown-Grant 1961). What role does iodide play in animal cells? In
previous works Venturi et al. (2000a, b) reported that it is possible to
hypothesize on the basis of the phylogenesis and embryogenesis three ways
of action of iodine:
1) An ancient and direct
action, on endodermal fore-gut, stomach and on ectodermal epidermis, where
inorganic iodides probably act as antioxidants.
2) A more recent and direct
antioxidant action, on salivary glands and mammary glands, thymus, ovary
and on nervous, arterial and skeletal systems.
3) A more recent indirect
(but also via non-genomic effects) action of the thyroid and its iodinated
hormones, on all vertebrate cells, which make use of specific
iodine-compounds: T4 and T3, which act in very small quantities and
utilize T3-receptors. Indeed thyroid hormones contain less than 1/30 of
total iodine amount.
Data from Wolff (1964), Evans
et al. (1966), Goethe (1999) and Venturi et al. (1985, 1999, 2000a, b,
2003) suggest that both these actions of iodine may still have an
important place in the cells of modern vertebrates. Broadhurst et
al.(2002) and Cunnane (2005) suggested that early Homo sapiens, living
around the Rift Valley lakes and up the Nile Corridor into the Middle
East, received iodine and n-3 fatty acids from littoral food resources.
Dobson (1998) suggested that Neanderthal man suffered I-deficiency
disorders caused by inland environment or by a genetic difference of his
thyroid compared to the thyroid of the modern Homo Sapiens. I-deficient
humans, like endemic cretins, suffer physical, neurological, mental,
immune and reproductive diseases. Iodine has favored the evolution of the
nervous system for a better adaptation to terrestrial environment as
recently reported by Cunnane (2005), who suggested that “iodine is the
primary brain selective nutrient in human brain evolution.” Cordain et
al.(2005) recently reported that the significant changes in diet, that
began with the introduction of agriculture and animal husbandry
approximately 10,000 years ago, occurred too recently on an evolutionary
time scale for the human genome to adjust. In conjunction with this
discordance between our ancient, genetically determined biology of
hunter-gatherers and fisher-gatherers human societies and the nutritional
patterns of contemporary Western populations, many of the so-called
diseases of civilization have emerged. Cordain suggests that iodine
deficiency was probably one, among other dietary variations, introduced
during the Neolithic and Industrial Periods, which have altered crucial
nutritional characteristics of ancestral human diet (simultaneously fiber
and polyunsaturated fatty acid contents). The evolutionary collision of
our ancient genome with the nutritional qualities of recently introduced
foods may underlie many of the chronic diseases of Western civilization.

Fig. 5. Daily dietary intake
of iodine, according to Food and Nutrition Board, Institute
of Medicine, 2001. Note that
an optimal iodine intake of 6.0 mg for breast has been
reported recently by Kessler
in °Breast J. 2004, 10:328-36
In 1883, Kocher observed that
atherosclerosis, a suspected ROS-caused disease, frequently appeared
following thyroid extirpation and suggested that hypothyroidism may be
causally associated with atherosclerosis. In 1930-50’s, potassium iodide
was used empirically in patients with arteriosclerosis and cardiovascular
diseases by European physicians (Cann 2006), and Turner (1933a, b)
reported the efficacy of iodine and desiccated thyroid in preventing the
development of atherosclerosis in rabbits. The antioxidant action of
iodides has also been described in brain cells of rats (Katamine 1985) and
in the therapy of some human chronic diseases of cardiovascular and
articular systems (Winkler et al. 2000). Liu (2000a, b) showed that ROS
and lipid peroxidations increase in I-deficient rats and children.
Recently, Aceves et al. (2005) and Cann (2006) suggested that iodides seem
to have preventive effects in breast cancer and in cardiovascular
diseases. Iodine seems to support anticarcinogenic defense of the
organism. Some data evidenced that iodine can prevent breast and gastric
cancer in humans (Eskin 1970, 1977; Venturi 2000b, 2001; Funahashi et
al.1996, 2001; Smyth 2003a, b; Kessler 2004; Szybinski et al. 2004; Abnet
et al. 2006 ). Aceves et al. (2005) reported that “Iodine is a gatekeeper
of mammary gland integrity” and proposes that an iodine supplement should
be considered as an adjuvant in breast cancer therapy. In the same
I-deficient territories human, animal and plant pathologies by
I-deficiency frequently coexist. Many researchers reported that Tasco-Forage,
an (iodine-rich) extract from the brown seaweed Ascophyllum nodosum, has
increased antioxidant activity and immune system in both plants and in
grazing animals ( Saker et al. 2001, 2004; Montgomery et al. 2001; Allen
et al. 2001; Fike at al. 2001). The evolutionary adaptation of terrestrial
vertebrates to environmental iodine deficiency has not finished yet (Cabello
et al. 2003), and most humans and terrestrial animals still need a dietary
iodine supplementation (Food and Nutrition Board, USA, 2001). According to
current W.H.O. statistics more than 3 billion people in the world live
nowadays in I-deficient countries. In the analysis of “National Health and
Nutrition Examination Surveys” data of moderate to severe iodine
deficiency is present now in a significant proportion (11.7%) of the U.S.
population, with a clear increasing trend over the past 20 years, caused
by reduced iodized table salt usage (Hollowell et al. 1998).
In conclusion, we suggest
that iodide acts as a primitive antioxidant and apoptosis-inductor with a
presumed anti-tumoral and anti-atherosclerotic activity. Studies of
molecular evolution of primitive antioxidants might provide the basis for
further research into “new” active substances against these pathologies.
|